Summary
In Bangladesh alone, over 25 million people suffer from chronic arsenic exposure. This exposure can lead to organ damage and can affect intellectual development in children. During this formative research experience, I learned advanced microbiology lab skills, such as how to use centrifuges, vortexers, gas cylinders, and sterile technique, to complete a series of anaerobic bioreactor experiments. The purpose of these bioreactors was to determine if bacteria from the gut microbiome can transform arsenic and if arsenic exposure changes the microbiome by changing the abundance of arsenic-related genes and of bacterial species.
Image by Md Arif Khan from Pixabay.
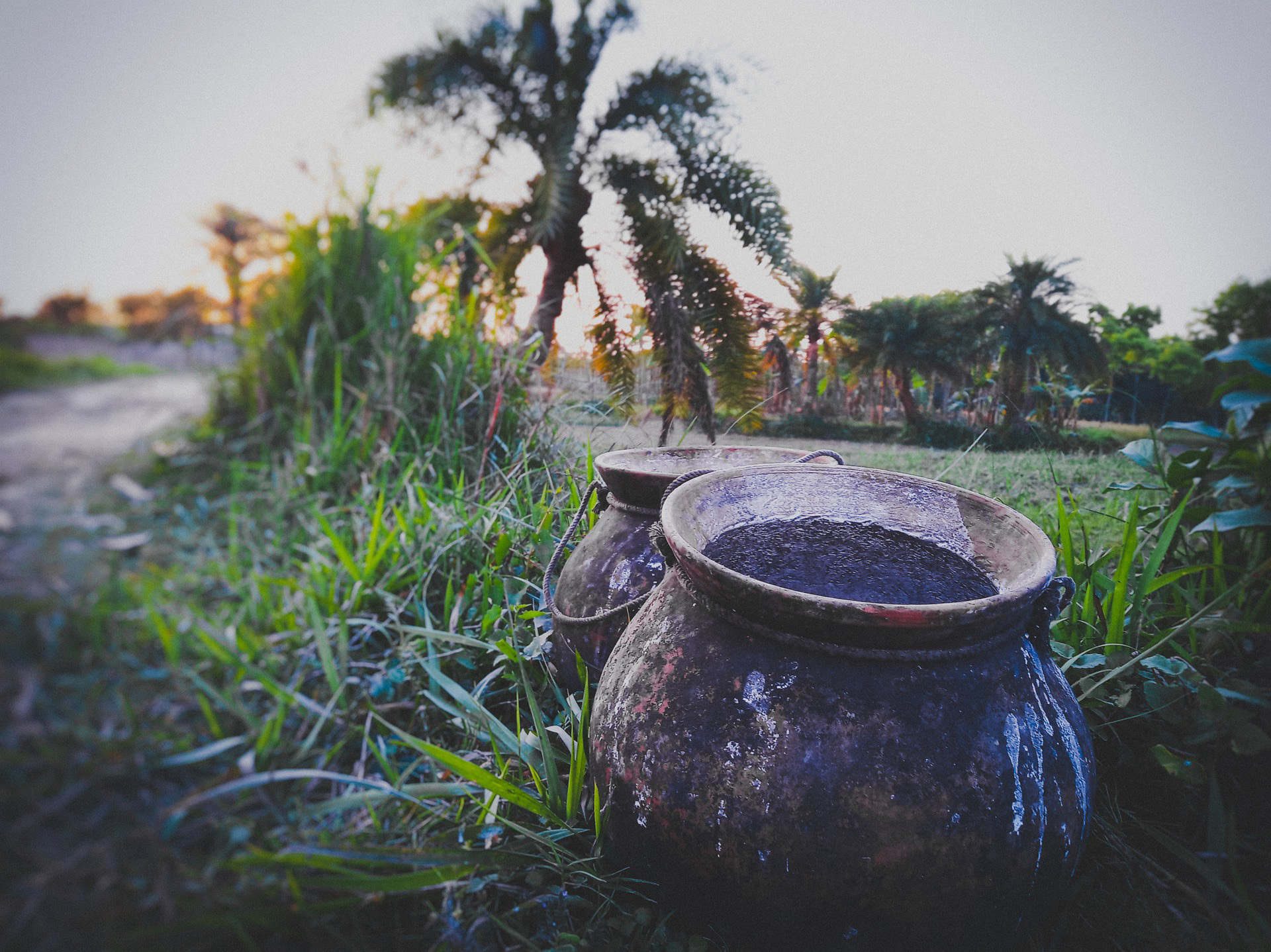
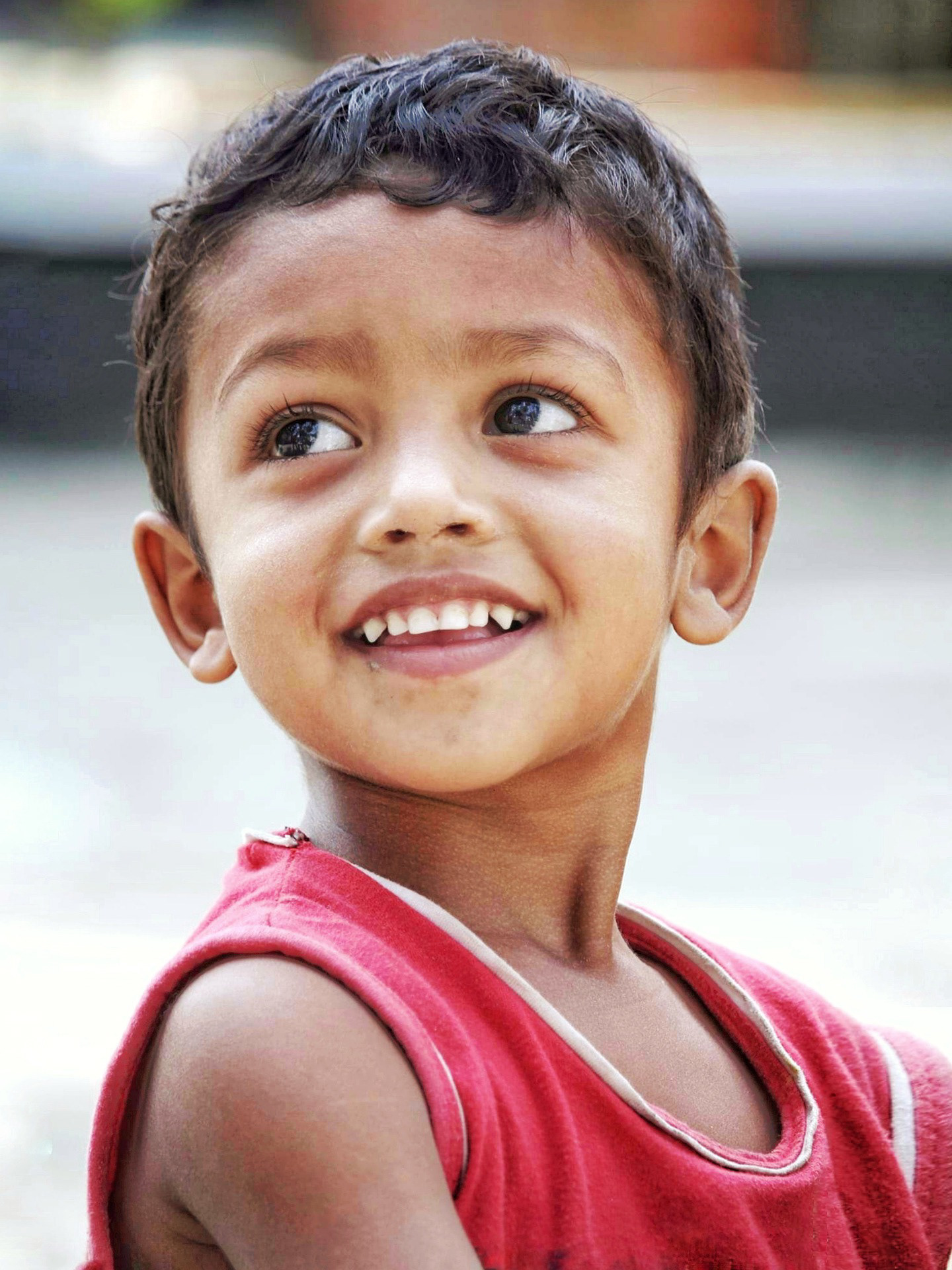
Motivation
Arsenic contamination of groundwater is a global public health concern. South Asian communities are particularly affected because many of the communities’ only sources of bacteria-free water are tubewells, which tap into arsenic contaminated groundwater 7-10 m below the surface. These communities not only drink the water, but also use it to irrigate crops. In Bangladesh alone, over 25 million people suffer chronic arsenic exposure from contaminated tubewells (1). This long term arsenic exposure can lead to arsenicosis, which can cause skin disease and internal organ damage (2-5). In children, chronic arsenic exposure can also affect intellectual development (6). Even if all the contaminated tubewells were replaced with access to clean water today, many would still suffer from the arsenic exposure they have sustained thus far (11). There are no effective treatments for chronic arsenicosis, so there is a need to begin developing strategies and therapies.
Populations with chronic arsenic exposure have shown variation in how each person metabolizes arsenic (7,8). One possible source of this variation is the gut microbiome, which plays an important role in digestion, metabolism, and immunity (9). Humans have individualized gut microbiomes with different microbes, strains, and genes (10). Our hypothesis is that consuming arsenic changes which microbes, strains, and genes are present in the microbiome, and that those differences contribute to variability in presence of arsenic-related disease.
Image by Abdullah Al Masud RAJA from Pixabay.
Objectives
During the months of March-December of 2016, my research objective was to contribute to the Arsenic Gut Microbiome Project by working on anaerobic bioreactors. The purpose of these bioreactors was to determine if bacteria from the gut microbiome can transform arsenic and if arsenic exposure changes the microbiome by changing the abundance and activity of arsenic-related genes and/or of bacterial species. My goal for the summer in particular was to perform bioreactor experiments using inocula from a control group of 10 healthy Stanford individuals. These data were later compared to bioreactors inoculated with samples from Bangladeshis with and without arsenicosis. I learned about and developed skills in: anaerobic experimentation and microbiology, experimental design, analysis of speciation data obtained by HPLC-ICP-MS, and analysis of DNA sequencing data. I also attended meetings with the Criddle Group to learn about other types of environmental engineering projects, design, and methods. This work provided an opportunity for me to further develop my research skills while contributing to a multidisciplinary project in environmental science and public health.
Images by me.
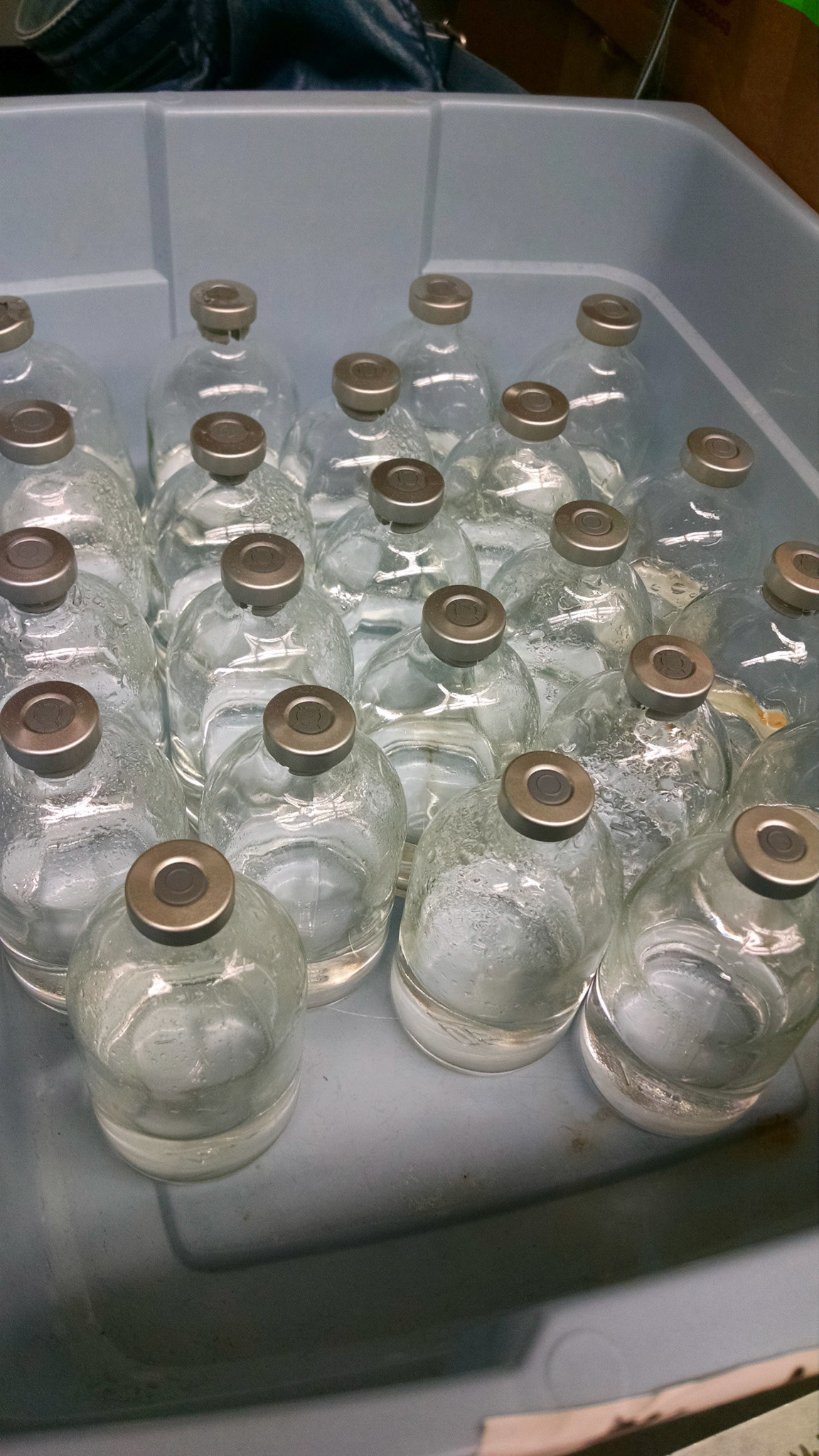
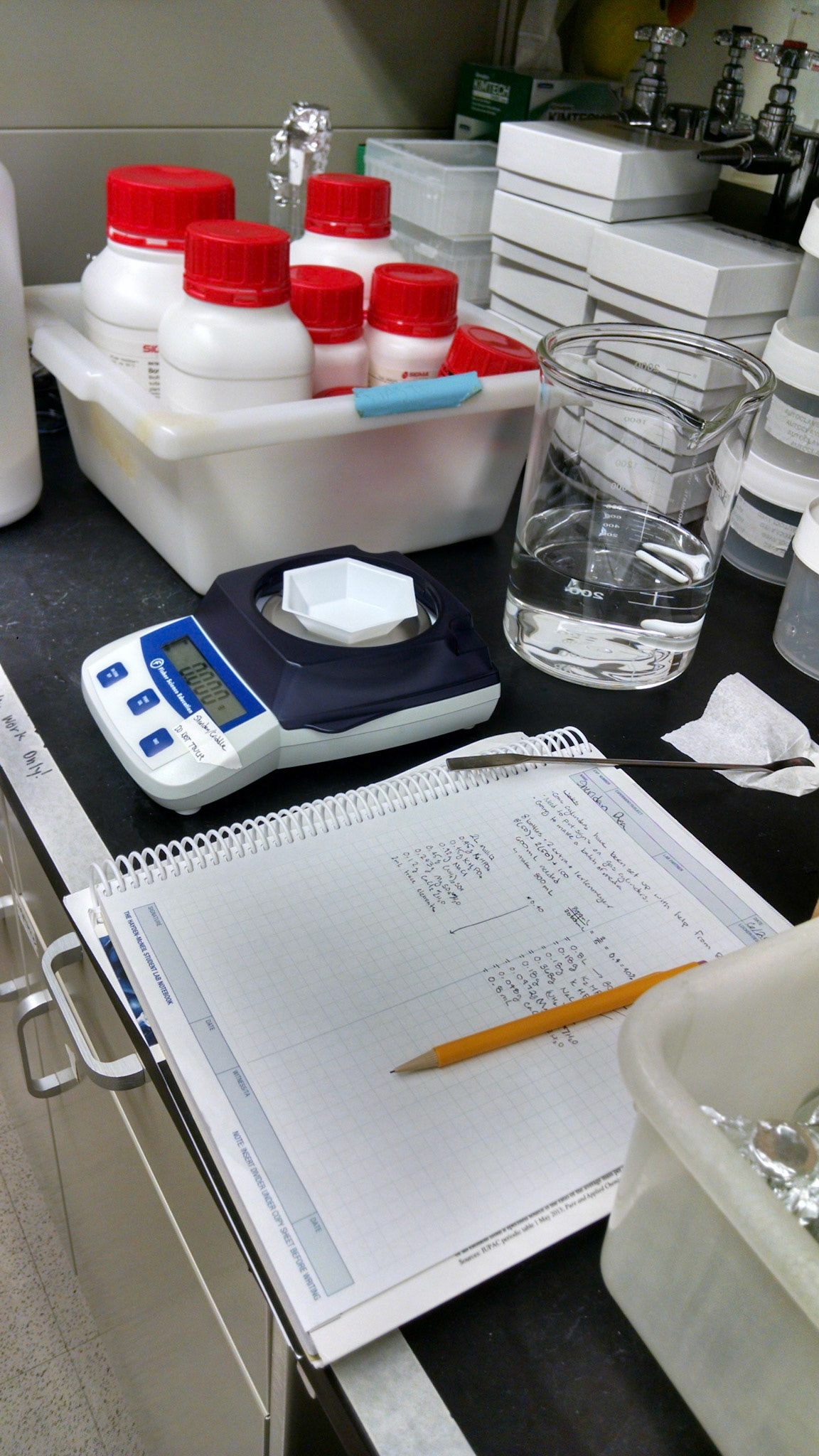
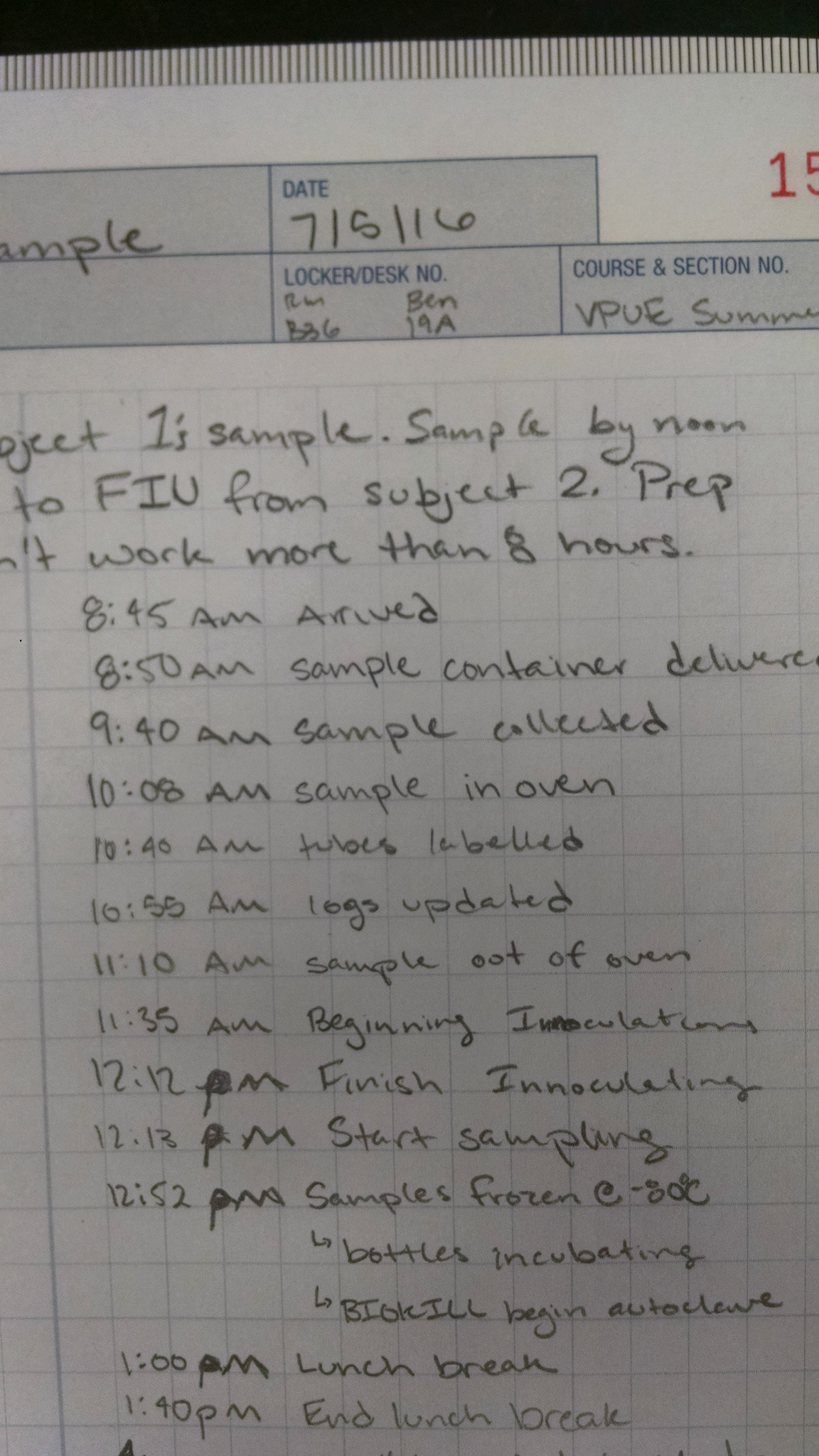
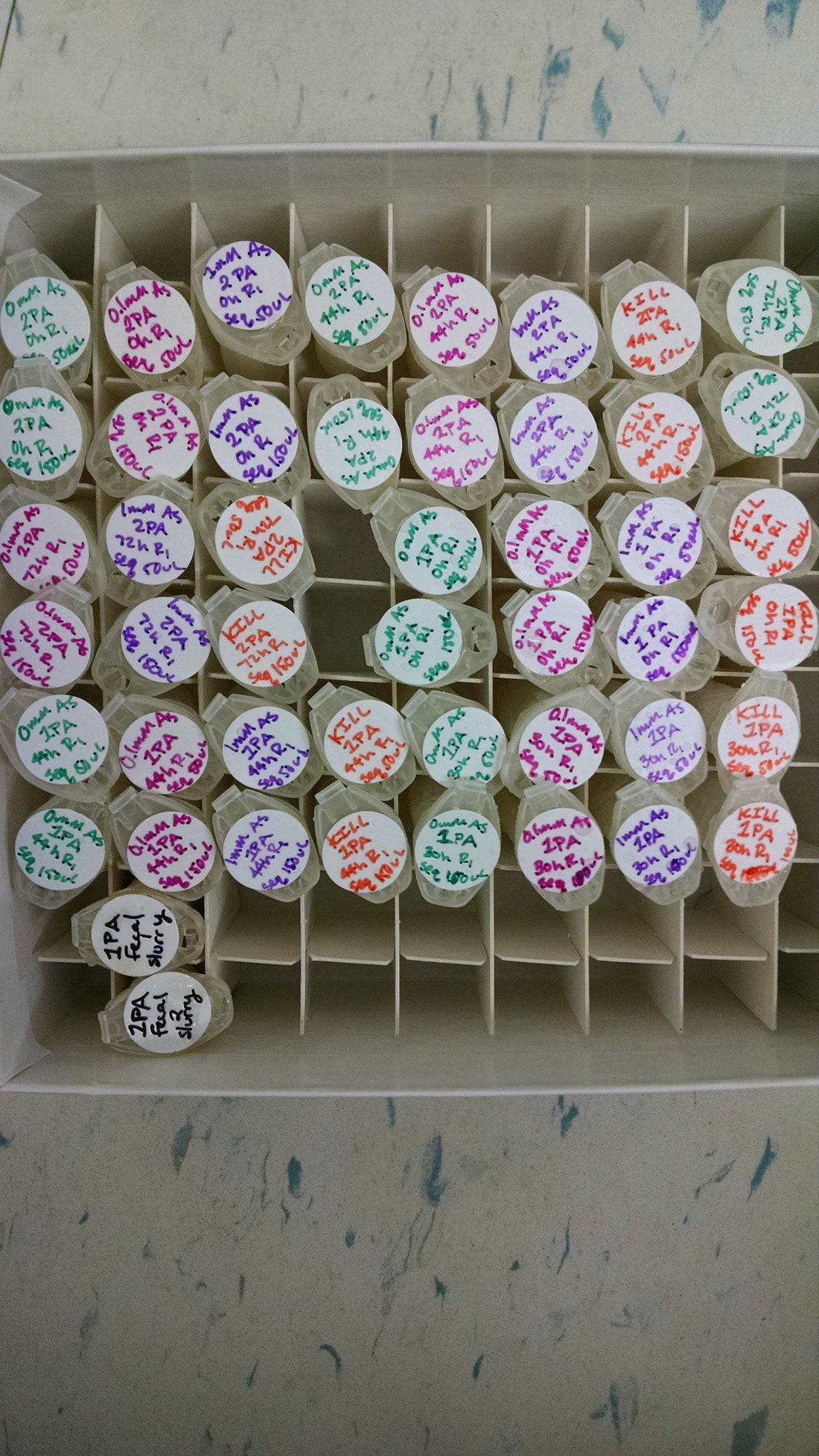
Procedures
The first step in the process of studying the relationship between arsenic and the gut microbiome is making media for the microbes to grow in. The media consists of salts, trace elements, vitamins, lactate, and is pH adjusted to 7.0. Then, sodium arsenate solution is injected into the sealed bottles to create the 4 different regimes: high arsenic (1 mM As(V)), medium arsenic (0.1 mM As), no arsenic, and a biological kill control (1 mM As(V))). Next, the bottles are inoculated with stool. The stool concentrations of the reactors are standardized by dry weight and have a final concentration of 10 g/L. The bottles are then incubated at 37℃ for 72 h. The incubation temperature and pH mimic the conditions in the gut, and the sampling time frame of 72 h is typical for times of ingested food/water in the human body. Over the 72 h period, the bottles are sampled at 0 h, 6 h, 20 h, 30 h, 44 h, 54 h, 68 h, and 72 h. Samples are stored at -80℃. Lastly, samples are prepared for arsenic speciation analysis via HPLC-ICP-MS and DNA sequence analysis via 16S rDNA sequencing and shotgun metagenomics.
Images by me.
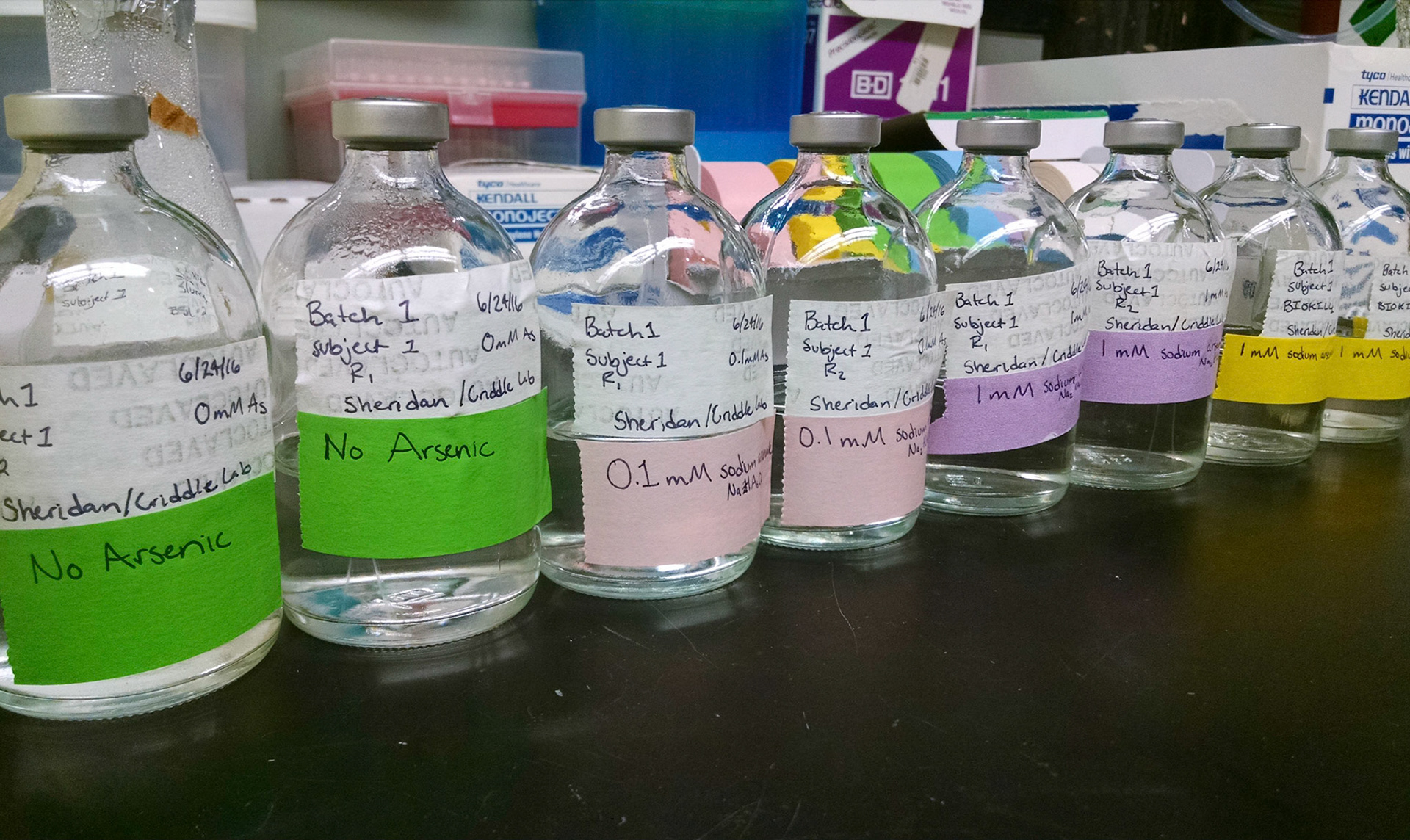
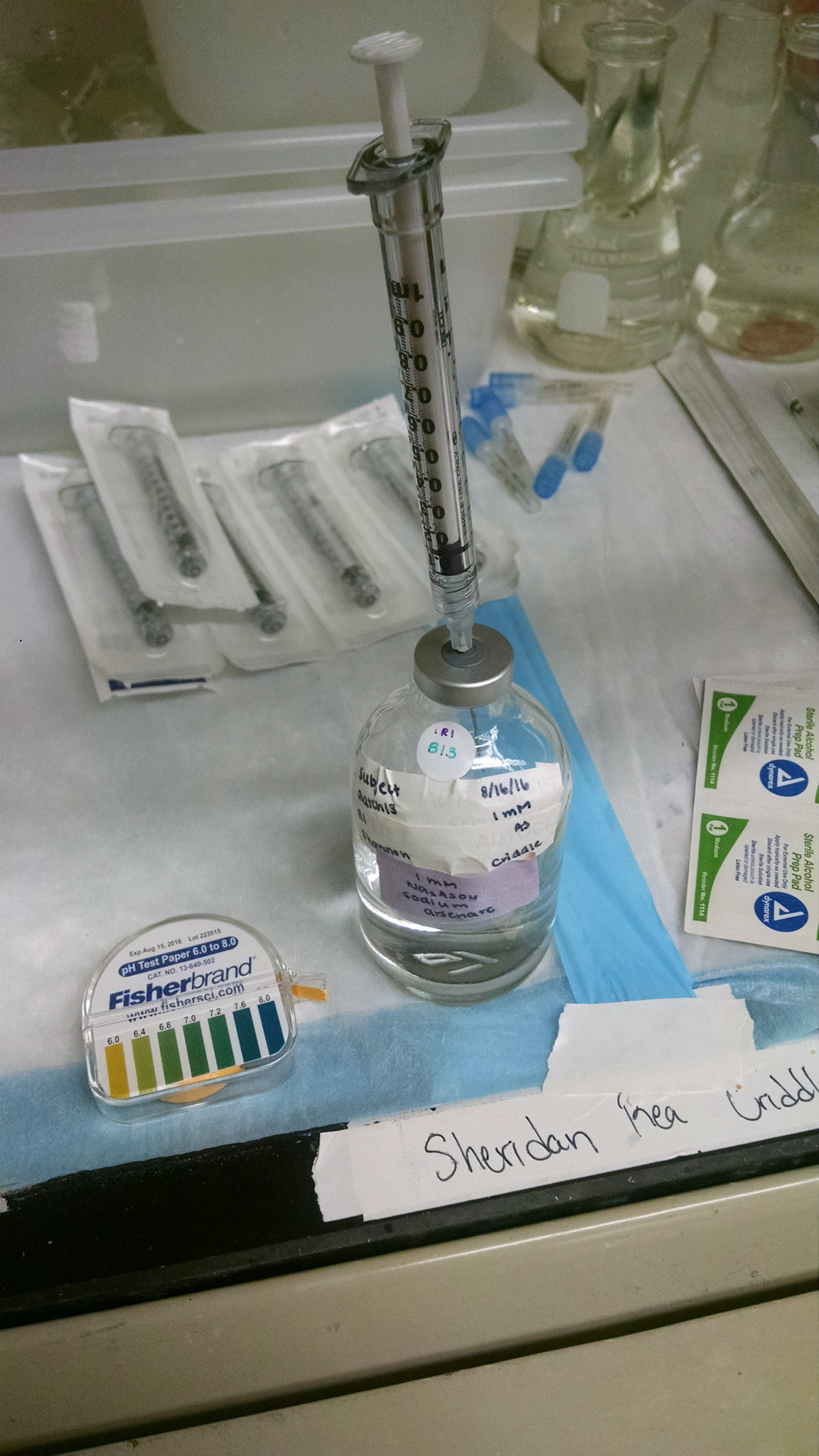
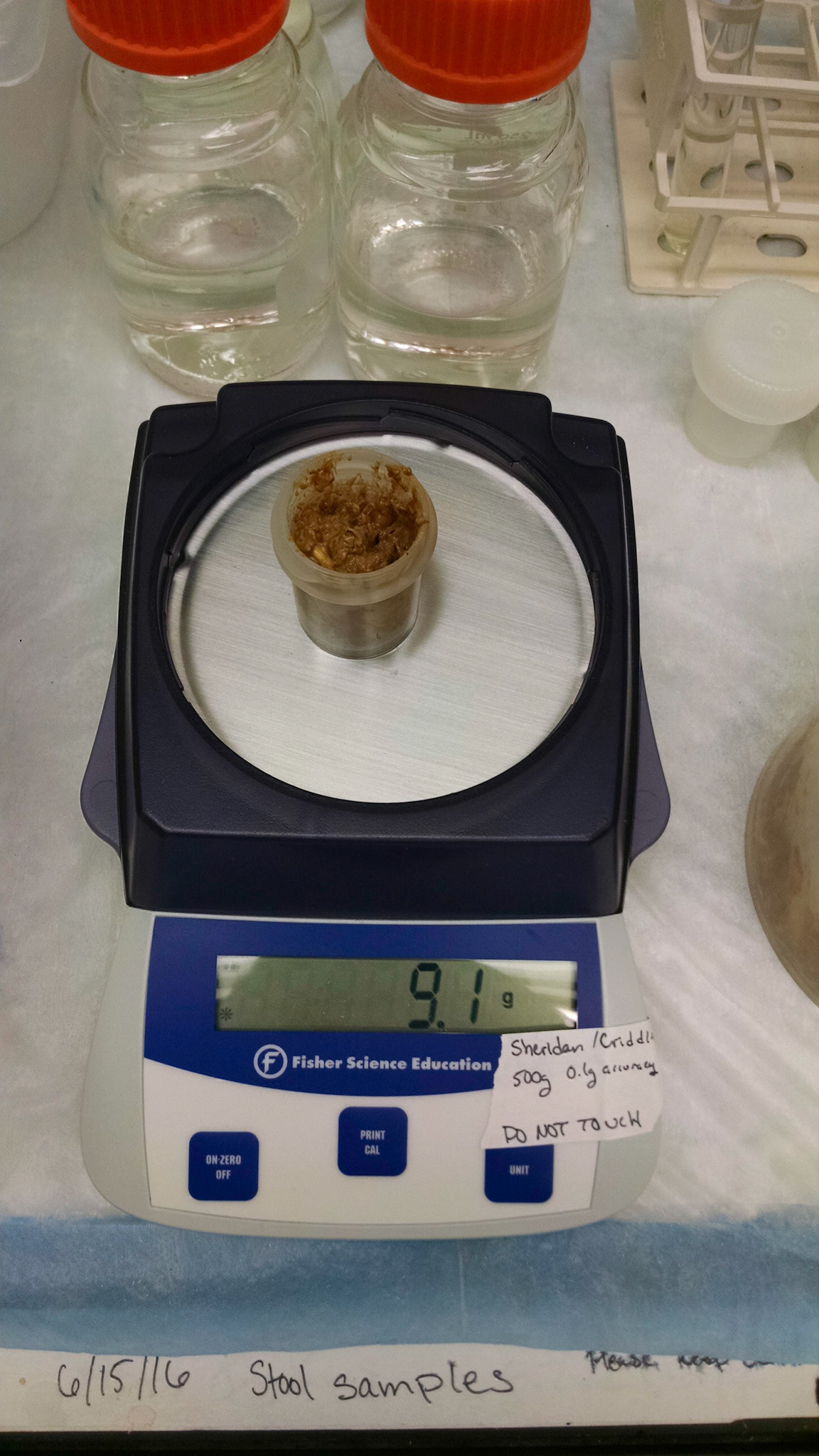
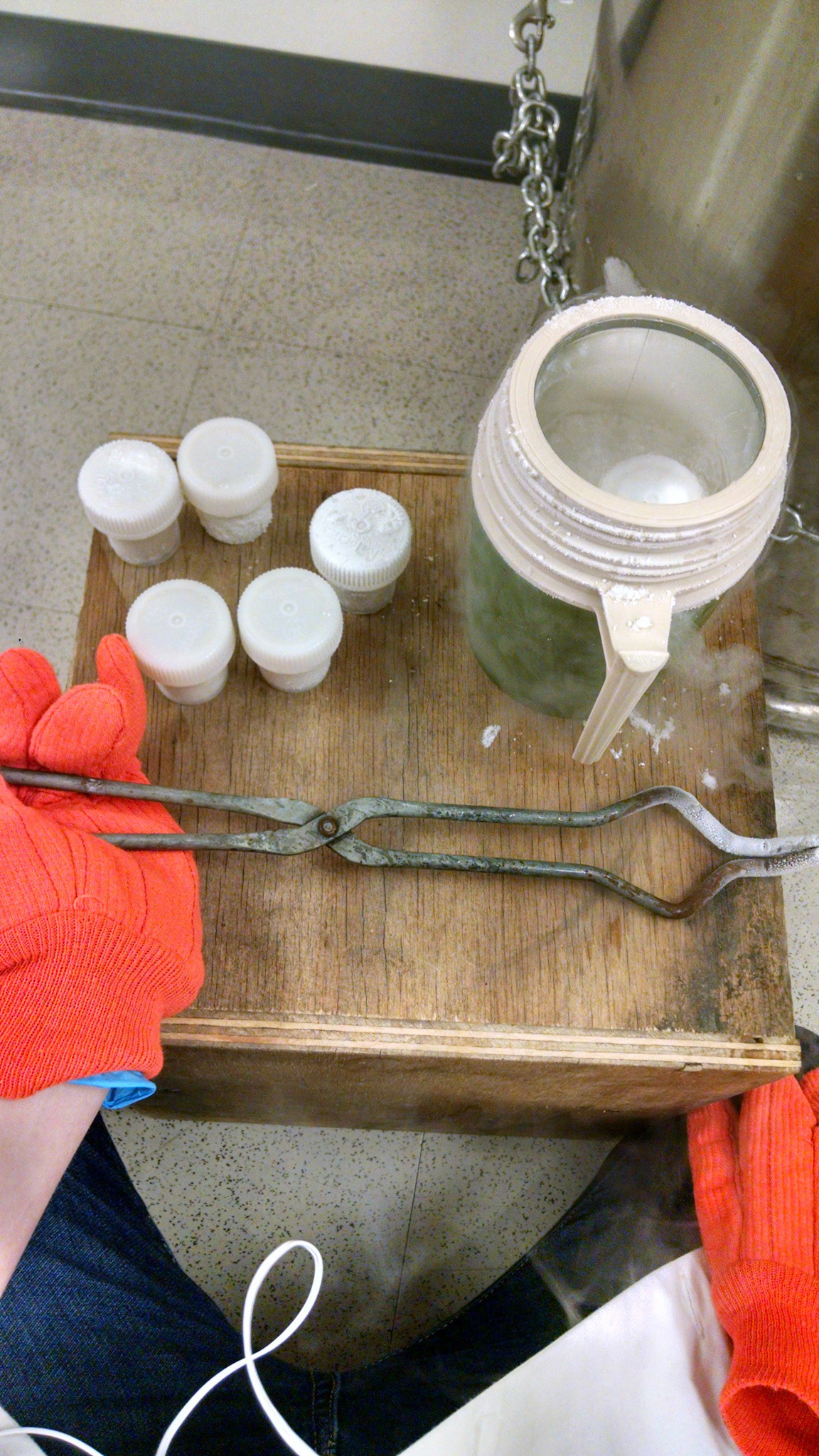
Major Outcomes
I started the summer having never used a centrifuge and came out understanding how to operate centrifuges, vortexers, gas cylinder connections, sterile technique, basic microbiology concepts, supply management, and general research methods, among other valuable skills. I was able to contribute to the optimization of the protocols and complete all 10 healthy Palo Alto subject experiments in addition to beginning experiments comparing fresh samples to flash frozen ones. Preliminary results from 2 subjects suggest that microbes of human gut origin are transforming the arsenic in the arsenic-inclusive reactors. At the end of the summer, I began learning how to analyze HPLC-ICP-MS arsenic speciation data and was able to finish these analyses by winter. In October I presented the preliminary results at the Stanford Symposium for Undergraduate Research and Public Service (SURPS). Perhaps the most important lesson I learned was the great amount of time, effort, and dedication research requires to produce good results.
Poster presented at SURPS in 2016.
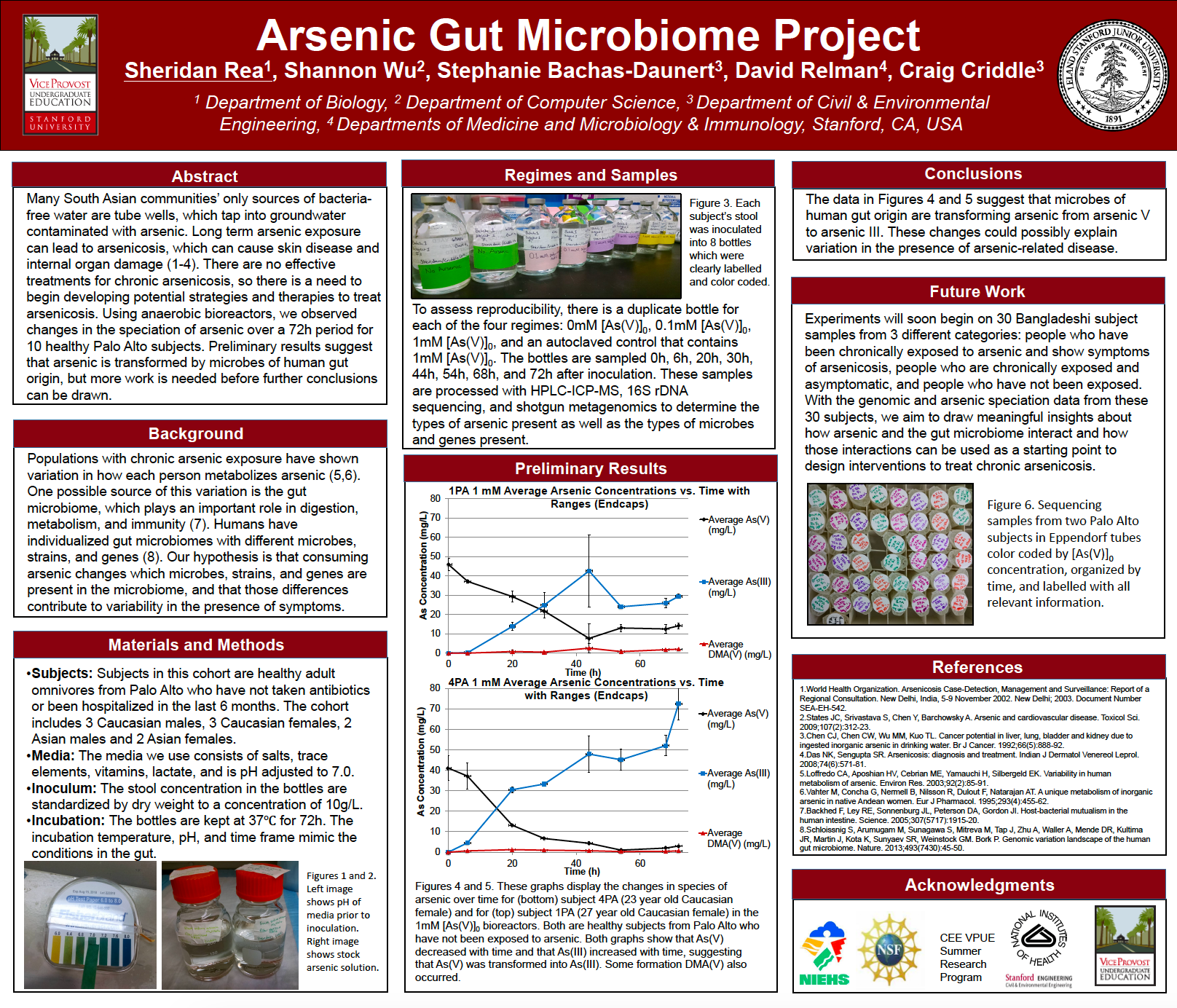
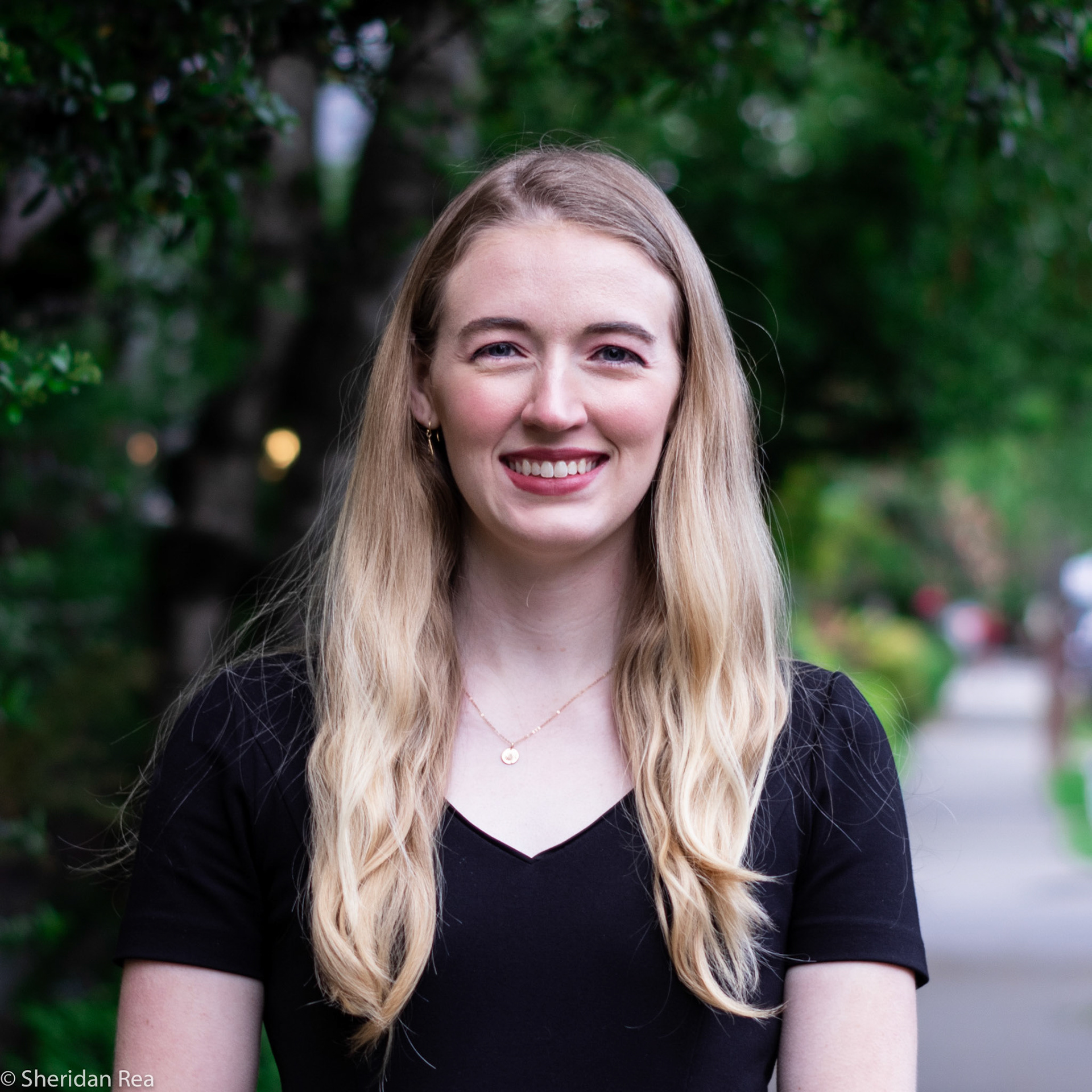
Sheridan Rea
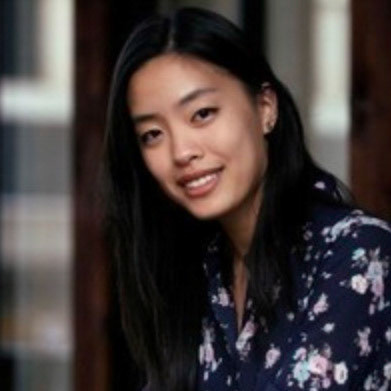
Shannon Wu
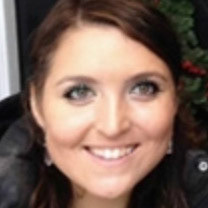
Stephanie Bachas-Daunert
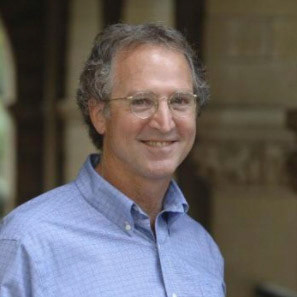
Dr. David Relman
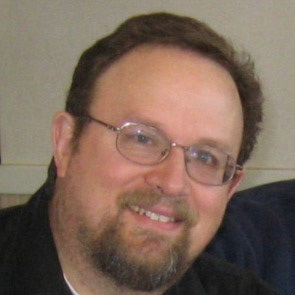
Dr. Craig Criddle
Works Cited
1. Mazumder DNG. Chronic arsenic toxicity & human health. Indian J Med Res. 2008;128(10):436-47
2. World Health Organization. Arsenicosis Case-Detection, Management and Surveillance: Report of a Regional Consultation. New Delhi, India, 5-9 November 2002. New Delhi; 2003. Document Number SEA-EH-542.
3. States JC, Srivastava S, Chen Y, Barchowsky A. Arsenic and cardiovascular disease. Toxicol Sci. 2009;107(2):312-23.
4. Chen CJ, Chen CW, Wu MM, Kuo TL. Cancer potential in liver, lung, bladder and kidney due to ingested inorganic arsenic in drinking water. Br J Cancer. 1992;66(5):888-92.
5. Das NK, Sengupta SR. Arsenicosis: diagnosis and treatment. Indian J Dermatol Venereol Leprol. 2008;74(6):571-81.
6. Wasserman GA, Liu X, Parvez F, Ahsan H, Factor-Litvak P, van Geen A, Slavkovich V, LoIacono NJ, Cheng Z, Hussain I, Momotaj H, Graziano JH. Water arsenic exposure and children's intellectual function in Araihazar, Bangladesh. Environ Health Perspect. 2004;112(13):1329-33.
7. Loffredo CA, Aposhian HV, Cebrian ME, Yamauchi H, Silbergeld EK. Variability in human metabolism of arsenic. Environ Res. 2003;92(2):85-91.
8. Vahter M, Concha G, Nermell B, Nilsson R, Dulout F, Natarajan AT. A unique metabolism of inorganic arsenic in native Andean women. Eur J Pharmacol. 1995;293(4):455-62.
9. Backhed F, Ley RE, Sonnenburg JL, Peterson DA, Gordon JI. Host-bacterial mutualism in the human intestine. Science. 2005;307(5717):1915-20.
10. Schloissnig S, Arumugam M, Sunagawa S, Mitreva M, Tap J, Zhu A, Waller A, Mende DR, Kultima JR, Martin J, Kota K, Sunyaev SR, Weinstock GM. Bork P. Genomic variation landscape of the human gut microbiome. Nature. 2013;493(7430):45-50.
11. Flanagan S, Johnston R, Zheng Y. Arsenic in tube well water in Bangladesh: health and economic impacts and implications for arsenic mitigation. Bulletin of the World Health Organization. 2012;90:839-846.